THE
WESTERN INSTITUTE
FOR
ADVANCED STUDY
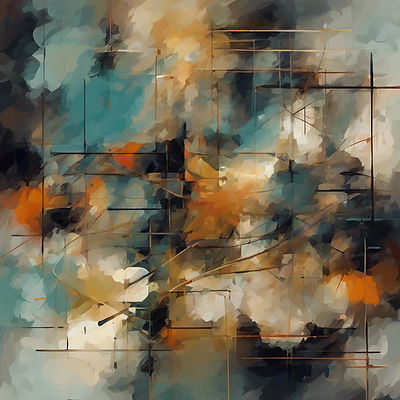
INFORMATION, COMPUTATION, AND MEANING
Our brains compute information, and we gain an understanding of the world.
At the heart of modern neuroscience and cognitive science lies a pressing question: How do we find meaning in the world, as our brains compute incoming sensory data and reflect upon that information?
This subject has roots in diverse fields, including philosophy, neuroscience, artificial intelligence, information theory, and perhaps even thermodynamics. It’s worth considering how these concepts—information, computation, and meaning—interrelate, drawing on insights from predictive processing, information theory, and neural computation to shed light on the brain’s role as an information processor.
Information, in its simplest form, is the reduction of uncertainty. Claude Shannon’s Information Theory provided a mathematical framework for quantifying information, which is now a cornerstone of modern communication systems. The brain, like any communication system, continuously receives, processes, and interprets information from the environment. But how does it handle this information, and what makes that information seem meaningful?
​
From a neuroscience perspective, the brain is a biological information processor. Every sensory stimulus, from watching TV to listening to music, can be viewed as data encoded in electrical signals traveling along neurons. The brain’s primary task is to make sense of this barrage of data, distilling meaningful patterns from raw sensory inputs. This process of information processing is not passive; it’s an active interpretation driven by prediction and computation.
​
Researchers have applied the principles of information theory to neuroscience, positing that the brain minimizes uncertainty (or free energy) by continuously making predictions and updating its model of the world based on sensory inputs [1,2]. As the brain receives information, it processes and computes the most likely interpretations, ultimately reducing uncertainty.
​
Cognitive scientists and neuroscientists alike have further explored how the brain computes information. The central theory is that the brain works as a computational machine, but it does so in ways that are vastly more efficient than any artificial system created to date [3,4].
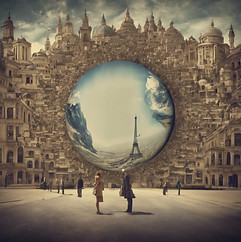
Computation in the brain isn’t just about number crunching; it’s about generating predictions based on available information and comparing these predictions to actual sensory input. The brain operates by hierarchical predictive coding, meaning that higher-level predictions about the world are constantly being refined by lower-level sensory input. When a prediction matches the incoming data, the system operates smoothly. When there’s a mismatch (a prediction error), the brain updates its internal model to better match reality (to make a better prediction). This feedback loop forms the crux of brain computation.
Meaning, in the brain, is therefore context-dependent and computationally derived from the patterns of information it processes. But how does raw information become meaningful?
​
Neuroscientists posit that meaning arises from patterns of prediction and feedback. According to predictive coding theories, information is interpreted by the brain as meaningful when it fits into pre-existing models of the world. Consider language processing: when you hear the word "dog," the brain immediately activates a cascade of associations, images of dogs, memories of past experiences, and expectations of what a dog might do. These associations are stored models in the brain, built from experience and refined over time.
​
Prediction errors, also play a role in the creation of meaning. When something doesn’t fit our model, like hearing a dog meow instead of bark, the brain flags this as a prediction error. However, the novelty of the experience often becomes the most meaningful aspect, leading to an update in the brain’s model – e.g. this is a humorous cartoon, not meant to reflect the actual state of the world, or this is a dog who has learned to imitate his longtime companion. In this view, meaning is dynamic and flexible, changing as our brains continuously refine their understanding of the world.
​
One of the most influential frameworks in understanding how the brain computes and generates meaning from information comes from information theory. According to Shannon’s model, information can be quantified as the reduction of uncertainty; the more uncertain the outcome, the more information is conveyed. This framework, when applied to the brain, suggests that meaning is based on consistency and correlation – an understanding of the gist of things, which reduces uncertainty in the brain’s predictive models.
​
The brain updates its beliefs about the world based on new evidence. Each time we encounter a new piece of information, we revise our understanding to better predict future events. The process of constantly updating predictions allows the brain to create meaningful interpretations of the sensory input it receives. Interestingly, some neuroscientists suggest that meaning in the brain isn’t just about understanding external stimuli but also about maintaining a balance between internal states and the environment. This idea is called the Free Energy Principle [5-6].
​
The Free Energy Principle posits that the brain is always trying to maintain a low-energy, low-entropy state, and it does so by minimizing prediction errors and surprise. In other words, meaning is not just about what’s happening outside the brain, but also about how well the brain’s internal models align with the external world to ensure survival and stability.
The brain’s computation of meaning also plays a crucial role in conscious experience. According to Global Workspace Theory and Integrated Information Theory, meaning is inextricably linked to consciousness. The theories propose that the integration of information across different neural networks creates a unified, conscious experience. In this view, meaning emerges from the global integration of information, enabling conscious thought, decision-making, and reflective awareness.
​
The interplay between information, computation, and meaning is also deeply tied to mental health. For instance, disorders like schizophrenia can be understood as disruptions in predictive coding. Friston and other researchers have suggested that schizophrenia arises from faulty inference mechanisms, where the brain incorrectly assigns high precision to certain predictions, making it difficult to distinguish between self-generated and externally generated experiences.
​
In summary, information, computation, and meaning are deeply intertwined processes in the brain. Information is the fundamental currency the brain uses to reduce uncertainty about the world, computation is the method through which it processes and predicts based on this information, and meaning arises from the successful integration of these predictions into a coherent model of the world.
​
From the early work of Shannon on information theory to the Free-Energy Principle and beyond, neuroscience continues to uncover how our brains handle the complexity of the environment with efficiency and purpose. These insights not only deepen our understanding of cognition but also open new pathways for treating neurological disorders and advancing artificial intelligence by mimicking the brain’s computational strategies.
​
And ultimately, the brain’s remarkable ability to process information, compute predictions, and derive meaning from this whole process is what allows us to navigate and understand the world around us - making sense of life's endless complexity.
REFERENCES
-
Melloni L, Schwiedrzik CM, Müller N, Rodriguez E, Singer W. Expectations change the signatures and timing of electrophysiological correlates of perceptual awareness. Journal of Neuroscience. 2011;31(4):1386-1396. doi:10.1523/jneurosci.4570-10.2011
-
Kok P, Jehee JFM, De Lange FP. Less is more: expectation sharpens representations in the primary visual cortex. Neuron. 2012;75(2):265-270. doi:10.1016/j.neuron.2012.04.034
-
Stoll EA. An energy-efficient process of non-deterministic computation drives the emergence of predictive models and exploratory behavior. Frontiers in Cognition. 2024;2. doi:10.3389/fcogn.2023.1171273
-
Stoll EA. A thermodynamical model of non-deterministic computation in cortical neural networks. Physical Biology. 2023;21(1):016003. doi:10.1088/1478-3975/ad0f2d
-
Friston K., Kilner J., and Harrison, L. A free energy principle for the brain. J. Physiol. 2006; 100: 70–87. doi: 10.1016/j.jphysparis.2006.10.001
-
Friston K. & Kiebel S. Predictive coding under the free-energy principle. Philos. Trans. R Soc. Lond. B 2009; 364: 1211–1221. doi: 10.1098/rstb.2008.0300